William T. Powers
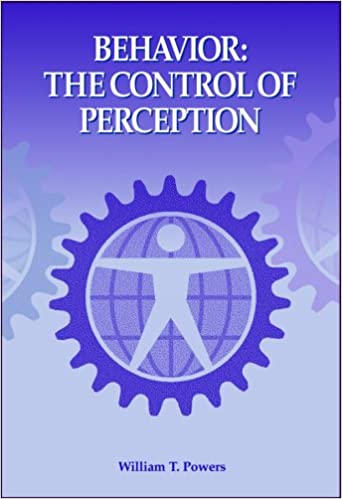
Preface |
This book represents, I hope, a step on the path back to a concept of man as autonomous, and away from the concept of man as automaton. Yet in allowing my humanistic bias to hold sway, I do not think I have denied science. Indeed, to most readers the first part of this book will seem a direct denial of my hope, for it gives a deliberately and specifically mechanistic picture of how the central nervous system behaves.Only after the mechanistic model is thoroughly understood will the reader see that it leads beyond ordinary mechanism and that it is capable of describing the interface between what we can represent as mechanism and what we cannot yet represent at all, but only experience.The traditional arguments between mechanists and humanists are represented by the dispute over purposiveness in behavior. The mechanists have argued that since organisms are made of matter, they are subject to the same determinism as any physical system, living or not, and in particular are bound by the cause-effect laws governing the behavior of matter. Hence we have behaviorism, which treats input as cause and output as effect and all that lies between as an automatic machine having properties but no purposes. The humanists have denied this picture on intuitive and subjective grounds, claiming that no machine can experience its inputs as well as respond to them, or conceptualize its own existence, or see the need for building a machine.This book presents an approach which may bring together these apparently irreconcilable points of view. The conclusion we are led to by the thinking in this book is that there is mechanism in behavior—but it is not the mechanism the behaviorists have in mind, for it is capable of having inner purposes in the full humanistic sense. On the other hand we are led also to seek not just a model of behavioral mechanisms, but a deep awareness that we are constructing a model; and we are encouraged to apply the model to ourselves. This process puts experience before theory but paradoxically shows that much that seems uniquely human is after all only acquired mechanism. The human remainder, the factor distinguishing man from animal or machine, is visible in the model only as a ghost, through its transcendent effects on the model itself.Is that the Soul of which I speak? The Atman? The Awareness? Of course it is. It is myself, yourself. But I have not been forced by this theory to conclude that this factor, this self, has to be treated either with tact or with reverence. It is a perfectly natural part of the totality we call a human being. It has functions in this otherwise mechanistically representable structure, and is not just along for the ride. Animals may have it, too. If it does not seem subject to the laws of physics, I take that as evidence that physics is still in an early stage of development. Whatever its nature, and I am sure it has a nature, it is adequately understandable through its effects on experience—and, incidentally, on learning. Adequately, that is, for any purpose I can now conceive.Scientists who view progress as a series of narrow escapes from meta-physical traps may find this book tough going in places, especially those places where I cheerfully give in to the temptation to anthropomorphize. It is precisely that kind of scientist who has the most to gain by understanding this book. If I anthropomorphize, be assured that I have done so with reasonable care and for a purpose. It is necessary, in order to understand the organization of human behavior, to recognize that one is constrained by any property he would impose on his experimental subjects if they too are human. What is necessary is not to avoid attributing human properties to human beings, but to avoid attributing such properties arbitrarily. There is at least one method, which is fully described later in the book, for testing the appropriateness of anthropomorphisms.It is a long way through this book, both in words and in new concepts. To save the reader a long wait for what will never appear, I should state now that the theory nowhere predicts how a particular person will react to a particular event. I have not been concerned with the kind of theory which predicts that event A will probably lead to behavior B. To me such theories are not theories at all, but summaries of observations. They in no way answer the questions I have been interested in, such as the question of what kind of organization is required to permit a person to reproduce in present time an experience contained in his present-time memories of the past. Not a particular experience—any experience.In that same vein, I have not been concerned with relating behavior to antecedent events. Rather, the central problem has been to find a plausible model which can behave at all. This has required a long effort to penetrate beneath surface descriptions to see what is being taken for granted. For example, it will be shown later that the brain does not command the muscles to act. That concept implies properties that the neuromuscular system simply does not have. It ignores the fact that we move about balanced on jointed sticks supported only by flabby rubber bands of variable tension. There is just no way the brain can select a muscle tension that will produce one and only one behavioral effect, even if that tension is accurately produced.The result of this approach is a model nearly devoid of specific behavioral content. I once felt that it was my duty to supply the model with content as well as form, but I am wiser now, and much more impressed with my ignorance. What is up to me is in this book. What I do best is in this book. Others who know more about behavior and many other subjects are the ones to put the content in. Where I have tried to make the form more comprehensible by suggesting content I have stepped over the bounds of my knowledge and have probably made mistakes. I trust that they can be corrected without obscuring what is most important about this model—and that is this: Behavior is the process by which organisms control their input sensory data. For human beings, behavior is the control of perception. That is what is important about the model—that, and all that is implied by it.—William T. Powers, 1973 AcknowledgmentsThe book you hold is essentially the same one published in 1973 by Aldine, then Aldine-deGruyter, which kept it in print for 30 years. I give them my gratitude for their long support, and thanks for gracefully complying with my request to accept other publishing opportunities—and wishing me luck when they did it. I have not updated the book other than making a few minor passages clearer; there are other newer publications for that (see the Bibliography). The main change is the inclusion of the chapter, Emotion, which was omitted from the Aldine/Aldine deGruyter editions.Let me also give my thanks to the researchers in the field of perceptual control theory (PCT’), as it is now called, who have integrated PCT into their work in a variety of disciplines. It is with a great sense of satisfaction, too, that I acknowledge the Chinese translation of B:CP by a group of scholars in the fields of Sociology and Philosophy who with this undertaking introduce the theory to the academic community in China.—William T. Powers, 2005 |
The Dilemmas of Behaviorism |
Since this book represents a break with traditional psychologies, it is appropriate to begin with a close look at behaviorism, the branch of psychology begun in America by John Broadus Watson near the start of this century. While many psychologists overtly reject the mechanistic formalisms of behaviorism, they adhere almost universally to its basic concepts and methods in one way or another. A humanistic psychologist, for example, may reject the idea that painful stimuli act on a passive nervous system to cause an organ to secrete adrenaline, but he may be perfectly willing to say that stress acts on a person to make him anxious. Even when stimulus and response do not show up in such disguises, it is widely agreed that a valid experiment requires that one establish fixed experimental conditions, manipulate a single variable, or several, and observe the consequent effects on behavior. Running throughout psychology (and almost identical to what is termed “scientific method” in psychology) is a particular concept of cause and effect. The cause, the immediate physical cause of what an organism does, lies outside that organism; the best the organism can do is to modulate the connection from the stimulus that is the cause to the behavior that is the effect. Those psychologists who find this point of view at variance with their own appreciation of human behavior simply do not compete at that level of discourse; they tend to withdraw from “scientific” psychology and to turn to humanistic and clinical pursuits. |
Models and Generalizations |
The conceptual collapse of behaviorism came about only in part because nature failed to fit the premises. Another kind of conceptual error has caused difficulties in behavioral research, paradoxically through failure to adopt the methods of physics. There is in psychological research (and many of the other “soft” sciences) what I consider to be a misunderstanding of the way in which physics has constructed theories, especially its classical theories.It is often taught in elementary science that theorizing is a process of generalization from specific observations. The term generalization, however, is too general, for it can be used to refer to three quite different strategies. It can mean a process of extrapolation, through which one extends a series of observations a short way into the future; it can mean abstraction, the classification of specific observations into categories; or it can mean model building, which I intend here to mean the interpretation of specific observations as consequences of hypothetical underlying causes. Psychology has been built almost exclusively on the first two senses of generalization, whereas the greatest successes of physics have come from use of the third.I propose to use the third method, the method of model building, in this book, which means that the behavioral theory to be found here will be different in nature from customary theories in psychology. The purpose of this chapter is to make clear the difference between my approach and that of most other theorists in psychology and similar disciplines. |
Premises |
One man, John Von Neumann, was largely responsible for the two major classes of modern automatic computers, analog computers and digital computers. His first “differential analyzer” was a mechanical contraption that solved simultaneous differential equations with pulleys, cables, levers, and balls rolling on disks. His first “stored program digital computer” was by today’s standards also crude. Nevertheless, both inventions have profoundly affected modern engineering and science—and modern theorists.THE DIGITAL-COMPUTER PREMISEAn unfortunate coincidence caused almost every theorist following Von Neumann to select the digital computer as the basic model of nervous-system activity. The coincidence was the fact that nerves behave in an all-or-none fashion: They generate an impulse or they do not, depending on whether the stimulation is above or below a threshold level. The comparison with the binary on-off elements of a digital computer was obvious, and was immediately taken up as the basis for a huge amount of theoretical effort.McCulloch’s (1972) nerve-net analysis was based on this concept; so was Wiener’s “stationary time series” analysis in Cybernetics (1948). Many others have continued in this tradition, and the mathematical theorems have multiplied and prospered. The main difficulty with such |
Feedback and Behavior |
Now that the general outline has been established for the brain model that is part of this theory, two last steps remain to be taken before the model itself can be developed: (1) an analysis of the role of feedback as objectively observable in behavior, and (2) an introduction to the internal structure and properties of negative feedback control systems; that is, the inner organization of such systems that explains the outward appearance of their interactions with their surroundings.THE FACT OF FEEDBACKWhat an organism senses affects what it does, and what it does affects what it senses. Only the first half of that commonplace observation has been incorporated into most psychological concepts of nervous system organization. The effects of behavior in altering subsequent stimuli, and even in directly causing stimulation, have certainly been noticed, but there has as yet been no correct analysis of this in any fully developed psychological theory.The most common treatment of the effects of an organism’s actions on the stimuli affecting it is to carry out the analysis stepwise: First, a stimulus causes a response via the organism, then the response causes a new stimulus or modifies the next stimulus, and the cycle begins again. As Hebb (1964) puts it, “Any behavioral response to a single stimulation thus produces a sensory feedback which can act as the initiator of a second response, whose feedback initiates a third response, and so on (p.58).” |
The Control-System Unit of Organization |
The concepts of the previous chapter constitute a conceptual model of behavior, similar in form and nature (although not in content) to many other psychological models. In this chapter a model of the behaving system will be developed in order to account for these objectively observable phenomena.System models are common in engineering but not in behavioral or social science. Outside of engineering the term system often appears to mean a network of disembodied relationships that link behaving entities together, whereas an engineer would probably translate the term to mean a diagram of the insides of a behaving entity. The difference is somewhat subtle, so before going on to develop the control-system model, let us first examine a diagram of the conceptual model we have just been through, the model describing the tracking situation (see Figure 5.1).The first fact to notice is the organism does not appear in this diagram. Every box in Figure 5.1 represents something that can be objectively determined by normal scientific procedures. The “muscle-tensions” box would be displayed as a feedback system if we allowed neurological data into the picture, but that would inevitably lead us deep inside the organism where this diagram no longer applies.There are, without the muscle-tensions system explicit, two feedback loops visible. Between the dashed lines is part of the stick-control loop. At the top is part of the spot-target relationship control loop. |
A Hierarchy of Control Systems |
The control-system model in the last chapter is a unit of organization. Behavior as a whole results from the operation of many such units at once. Many control systems may act simultaneously on the same environment yet remain independent—for example, one system might control the sum of two quantities while another simultaneously and independently controls the difference between them. Even when interactions occur (the control actions of one system tending to alter the controlled quantities of other systems), those interactions maybe treated as any ordinary disturbances are treated. Each system can simply adjust its own output to cancel such interactive effects. There is another mode of interaction, however, which we will now consider, a mode in which one control organization is part of a larger one and hence not at all independent of it.Figure 6.1 is a hierarchical model of the tracking situation that has been our central example so far. Since we are now definitely inside the behaving system, the muscle-tension control system can be explicitly included, so we have a three-level model.This complex-appearing diagram will unravel if approached systematically. Starting at the top, we accept as given a reference signal standing for the overall goal relationship of spot on target. The signal representing the actual relationship comes in from the left; it results from three steps of visual information processing. The resulting error signal enters the “output function,” a neural computer that derives the appropriate reference signal for stick position (as kinesthetically sensed) |
First-Order Control Systems: Intensity Control |
The central nervous system is bounded by a set of output devices, the muscles (and glands), and by a set of input devices, the sensory nerve endings. Everything outside a normally operating nervous system that can affect the flow of information into it must act by stimulating sensory nerves at its boundary; every effect the nervous system can have outside itself results from neural currents reaching its boundary from inside. A model of the behaving system is therefore a model of processes that take place inside the boundary, all else being the environment of the behaving system (even if sometimes contained within the same physical envelope).In this chapter we will define and analyze a set of negative feedback control systems that lie just inside the boundaries of the central nervous system. In effect, a second boundary will be defined, such that all control systems in this set lie inside the overall boundary, but outside the new boundary, in a shell completely surrounding (topologically) the remainder of the nervous system. When these first-order systems in this outer shell have all been accounted for, they will become the total environment which the remaining systems can sense and affect, for the first-order systems are the only means by which the inner parts of the nervous system can interact behaviorally with what lies outside the nervous system. That being the case, the only environment with which the inner systems can interact is the set of first-order systems. |
Second-Order Control Systems: Sensation or Vector Control |
We have now separated from the rest of the central nervous system a specific set of control systems concerned with the control of sensed effort-intensity, together with all sensory endings that are not involved in a first-order control system. This means we have accounted for all means whereby the central nervous system can produce forces affecting the environment outside the nervous system, and for all means whereby external physical quantities impinging on the nervous system give rise to sensory neural currents. Now we will look at the remainder of the central nervous system—the brain proper—to find another layer of control systems. The object will be to define a set of control systems that is hierarchically superior to the first-order systems, and which at the same time is as close to the level of first-order systems as possible. The general goal for the next few chapters will be to continue the process we have begun of isolating sets of control systems that in some way have similar relationships to the external world and to do so in such a way that all such systems, known or hypothetical, are accounted for by the definitions. The picture to be developed here is perhaps optimistically neat, but considerable evidence exists in support of at least the general relationships to be discussed as we progress from this new order of control, second order, to the highest order about which I am willing to venture even a guess, the ninth order. The general relationship proposed in this chapter between second-order control systems and first-order control systems puts the second- |
Third-Order Control Systems: Configuration Control |
Many perceptual signals from second-order systems split into two branches, much as the kinesthetic perceptual pathways divide when they enter the spinal cord. As in the first-order systems, the perceptual signals travel to comparators, here to complete the second-order control loop, and duplicates of these signals pass upward (inward in our concentric-shell visualization) to higher-order systems. The reference signals from higher-order systems directly control some of the second-order perceptual signals going to higher-order systems, and uncontrolled second-order perceptual signals also travel toward the inner parts of the brain.The pathways leaving second-order input functions, the sensory nuclei of the brain stem and other functionally similar structures, go to two nearby destinations: the cerebellum and the sensory nuclei of the thalamic region, the midbrain. In these two regions the sensation signals converge and enter another stage of information processing. From these same general regions come the reference signals for second-order systems. We may therefore expect to find third-order control systems in the cerebellum and in the thalamus and its immediate surroundings. (There is another more remote location in the cerebral cortex which I will discuss later.)The behavioral role of the cerebellum appears to concern a host of kinetic coordinations which are largely carried out without the need of, or apparently the possibility of, conscious awareness. Most of the |
Fourth-Order Control Systems: Control of Transitions |
From here on, as help from experiments with the brain diminishes, the development in this book will lean more and more toward finding plausible definitions. The emphasis will turn more toward an analysis of direct experience, that same world of appearances that is normally taken for granted in the design of scientific experiments. The uncritical acceptance of appearances and the unconscious assumption that perceived properties of the external world are objective properties is the basic reason for the lack of the brain experiments that would be most useful here. Hence, even though we may have to use general observational data and subjective perceptions, the result will point out experiments that need to be done and data that need informed reinterpretation.PERCEPTION OF CHANGESome parts of the brain responsible for the fourth order of control and perception must be between the thalamus and the inner layer of the cerebral cortex—of that we may be reasonably sure because, as we will see in the next chapter, there is good evidence for localization of the fifth order of control systems in this layer of the cortex, and circumstantial evidence seems to require an intermediate level of systems. (This does not rule out “misplaced” systems of fourth order.)The most elementary example of fourth-order perception is also the easiest to identify; without it, movies and television would not work. |
Fifth-Order Control Systems: Control of Sequence |
If we imagine that the perceptual signals of all levels through fourth order somehow can become objects of awareness, we can ask at this point what the world looks like to this brain. Movement and rate of change can be detected; there are objects and arrangements and static patterns that can be seen; each configuration decomposes into a variety of sensations; and with each sensation is associated some average degree of intensity. The organism can cause configurations of its own limbs to change at controlled rates, can maintain static configurations against disturbances, can control the magnitudes of sensations (which we sometimes call vectors), and can at least control the intensity of its own effort signals.What we have, however, is still a very elementary organism, no matter how complex its equipment for handling variables for these four levels may be. It can control its own position and some external configurations; it can control movement. Nothing more. It cannot, for example, select one movement instead of another; if any organisms exist with only four orders of control system, the reference signals for movement must originate either from stimuli, or from genetically transmitted and stored information. We cannot even say that this organism is skilled, for although it can make configurations change at controlled rates, it cannot select when or in what combination to produce these changes. |
The Brain’s Model |
The five levels of perception which have been more or less defined in the past few chapters consist of signals derived from the interaction of physical quantities and sensory endings through five successive steps. Under the basic postulate of perception (that all perceptions are neural signals and are all that is available to awareness), these signals constitute an analog model of the external world. Let us drop back to first-order input functions, to see what this means.A first-order input function can be defined as a set of sensory receptors which always respond at the same time (the most likely tie being physical proximity). The signal emitted by this first-order input function is produced by a physical quantity, and the magnitude of the neural current depends on the intensity of stimulation. Whatever the relationship, whether linear or nonlinear, it can be said that the neural current is an analog of the physical quantities.Analogs in the precise sense meant here, however, must always be stated so that it is clear what is an analog of what: a neural current that arises because of contact of the hand with an object is not an analog of that object; one neural current simply does not have enough degrees of freedom to represent every important aspect of the object. Rather, the neural current is the analog of the magnitude of the physical effects that are causing the neuron to fire, in this case the magnitude of pressure, or deformation of tissues.Saying that the neural current is an analog of an external quantity |
Higher Levels |
We now pass from classes of perception that can be seen as exterior to ourselves to those which seem to be inside ourselves—from the world of “physical reality” to the world of “subjective reality.” This model makes no special distinction between these subdivisions, however; they are matters of training, convention, and convenience as far as we are concerned here. All levels are “inside”; of what lies outside our sensory endings we know next to nothing. The level we are about to consider is a transitional one, for certain of its aspects seem easy to conceive of as aspects of the solid, real, lower-order world, while other aspects strike us more as conceptual in nature.SIXTH-ORDER CONTROL SYSTEMS: CONTROL OF RELATIONSHIPSConsider a typical behavioral experiment. Having learned to repeat a list of words, after an interval the subject is tested for recall. Later the same experiment is repeated, but this time a second list has to be learned between a new initial list and the later recall test. It is found that learning the intervening list interferes with recall of the initial list. This phenomenon is named retroactive inhibition, and takes its place as a scientific fact. Clearly the experiment consists of observable events in the sense of our model; the question is: What has been learned about an organization of higher level than events? Does this experiment help us construct another level in the model? Not in any obvious way.We could go on looking at other types of events in the hope of seeing the nature of the next level up. We could try experiments involving |
Learning |
Several types of phenomena are commonly subsumed under the term learning: I will call them memory, problem-solving programs, and reorganization. Using a computer model as an analogy, we can distinguish these phenomena from each other. A memory type of learning corresponds to typing a sentence which is received and stored by a computer and then on some preset signal is automatically typed out again. Any time the signal is given, the sentence will be typed, proving that it has been “learned.” However, the organization necessary for this operation has no relationship to what has been typed—it will work for any character string. This is rote learning and involves no modification of the system’s organization.Problem-solving programs are exemplified by the programs of Newell, Shaw, and Simon (Chapter 13). Programs are fixed lists of instructions (reference levels for lower-order systems in human beings) with choice points in the lists. Both memory and present-time inputs are important elements. Problem-solving programs can be very complex, and behavior can appear adaptive because changing input information can result in switching to new subroutines that cause drastic changes in the apparent character of the program. No actual changes in organization, however, take place: the same list of operations remains in use, and even the subprograms may retain the same organization. All that changes is the path followed through the network of contingencies, all possible paths being determined when the writing of the program is finished. |
Memory |
From the standpoint of a behavioral experimenter, memory is an exceedingly complex phenomenon with no certain physical basis. Not only can human beings repeat sounds they have just heard, but they can pronounce sounds on the basis of what they have just read, and repeat acts that they have seen others perform. Practically every regularity in behavior can be interpreted in some way as a memory phenomenon. Even the physical state of the organism can be seen to fit the most generalized definition of memory: present traces of past experience. Big muscles are memories of exercise.Too generalized a definition of memory, however, is of little use because such definitions melt indistinguishably into concepts like growth or performance. I think that we should deliberately forego the maximum possible generality, if only to give us something specific to talk about. Memory can be treated as a reasonably well-defined phenomenon, the storage and retrieval of information carried by neural signals. Under this definition, the structure of the learned hierarchy of control systems, although constituting a trace of past experience, would not be called “memory” because that structure itself is never represented in the form of neural signals. It carries neural signals, but is invisible to them.The previous chapter on learning dealt with long-term changes in behavioral organization resulting from changes in the effective structure of the brain. We have, therefore, already dealt with a large class of phenomena that is commonly studied under the label of memory, without once depending on the storage of information carried by neural |
Experimental Methods |
The theory in this book is based not on extrapolation or generalization but on model building as discussed in Chapter 2. The experiments which will test and improve the theory will therefore not rely heavily on statistics, but far more on determination of the properties of a system as measured in its behavior.The concept of measurement of properties is not entirely alien to psychology. In psychology there have been extensive studies of the physical correlates of vision, hearing, taste, and other sensations (order 2), the properties of neuromuscular behavior (including feedback-oriented studies of tracking) and other lower-order properties of behavioral systems in general. The lack of a coherent model of multiordinate behavioral organization, however, has prevented any of these approaches from coalescing into a study of the whole human system and has forced most psychological research into a dependence on statistics.My objections to the statistical approach to behavioral research, exposed here and there in previous chapters, are not based on a dislike of statistics per se. Rather they reflect my dislike of the blindness of statistical manipulations to organizing principles, and of the way statistical facts entice us to overlook the individual organismic properties that must underlie all statistical facts. There is a magical aura about statistical relationships. Certain events manipulated by an experimenter correlate with subsequent changes in behavior, with no causative links at all being visible: the relationship, for all anyone can tell, may in fact be magical. |
Emotion |
There are three major categories of sensations. One category is associated with what we learn to identify as events that take place outside the body; sensations of light, of smell, of sound, of temperature can arise or change without our taking any action at all. In control system terms, these are the quantities that seem especially susceptible to disturbance by outside agencies. Then there is a set of sensations that seem to be under more or less direct voluntary control: sensations of effort, of joint angle, of facial expression, of touch, of movement—these sensations hardly ever appear unless one wishes them to appear, and external events have very little power to affect them in comparison with our own control over them. The third category is odd in that it seems to have its location entirely inside the body, and yet at times seems to be under control by the external world, or by factors in ourselves over which we have no voluntary control at all: these are feelings or emotions. This chapter represents an attempt to bring the third category of experience into the scope of this model.Emotions are well-known to have two components, one thought-like and one sensation-like. Physiologists have long identified the sensation-like component as arising from changes in the biochemical state of the body-alterations in the levels of action of the major organ systems. In fact for all strong emotions, there appears to be a more or less common physiological state, termed the general adaptation syndrome. The adrenal cortex releases adrenaline into the bloodstream, causing a release of stored energy, constriction of the peripheral capillaries, an increase in heart-rate (and a rise in blood pressure), increase |
Conflict and Control |
In this final chapter we will look into applications of this model of human behavioral organization. The reasons for applying this model even in its present rudimentary state are twofold. First, the results are interesting and even useful in ordinary living; it is a theorist’s prerogative to think so. Second, this is the best way I know to combat the “nothing-but” syndrome. The second reason may be the most important for the future of this theory.The “nothing-but” syndrome is the demonstrated tendency of human beings to upgrade similarities to the status of identities. Thus I have heard that the reorganizing system is “nothing but” drive-reduction theory, that the control-system unit of organization is “nothing but” reafferent stimulation, that higher-order perceptions are “nothing but” concepts. Each nothing-but-er was attempting, often with generous intent, to find a niche for this control-system model in some established scheme. It is my present conviction that all such attempts will fail, not because this theory owes nothing to its predecessors (it owes everything), but because it carries us beyond its predecessors into new territory. It makes us think about behavior in new categories. Attempts to make this model congruent with older non-control-system concepts always, in my experience so far, require a misinterpretation of the properties of control systems or a direct contradiction of a postulate in the model. I do not object to justified contradictions, but only to accidental and thus indefensible contradictions. |
Appendix: Control System Operation and Stability |
This discussion is intended to acquaint the reader only with the most elementary aspects of control system theory—just enough to aid in appreciation of the basic rules of thumb about stable control systems. For more advanced information see any text listed under Servo-mechanisms or Control Systems.LINEAR ANALYSIS OF A CONTROL SYSTEMFigure A.1 is the standard control-system diagram used elsewhere in the book. Here, however, each relationship is considered quantitatively.The k-constants shown represent a linear approximation to the actual input-output relationships of the various functions involved. Thus for the input function, the constant ki relates the perceptual signal p to the input quantity qi : p = ki qi.The error signal e is taken to be equal to the difference between the reference signal r and the “perceptual” signal p. In actuality this is more likely to be a weighted difference, but the weighting factors are most conveniently absorbed into ki and ko. Thus e = r – p. The sign is chosen so that feedback will be negative. The output quantity qo depends on the error signal e according to qo = ko e. The output quantity qo and the input quantity qi are physical quantities and are expressed in physical units, whereas the signals p, r, and e are neural variables and must be expressed in signal-units (impulses per second). |